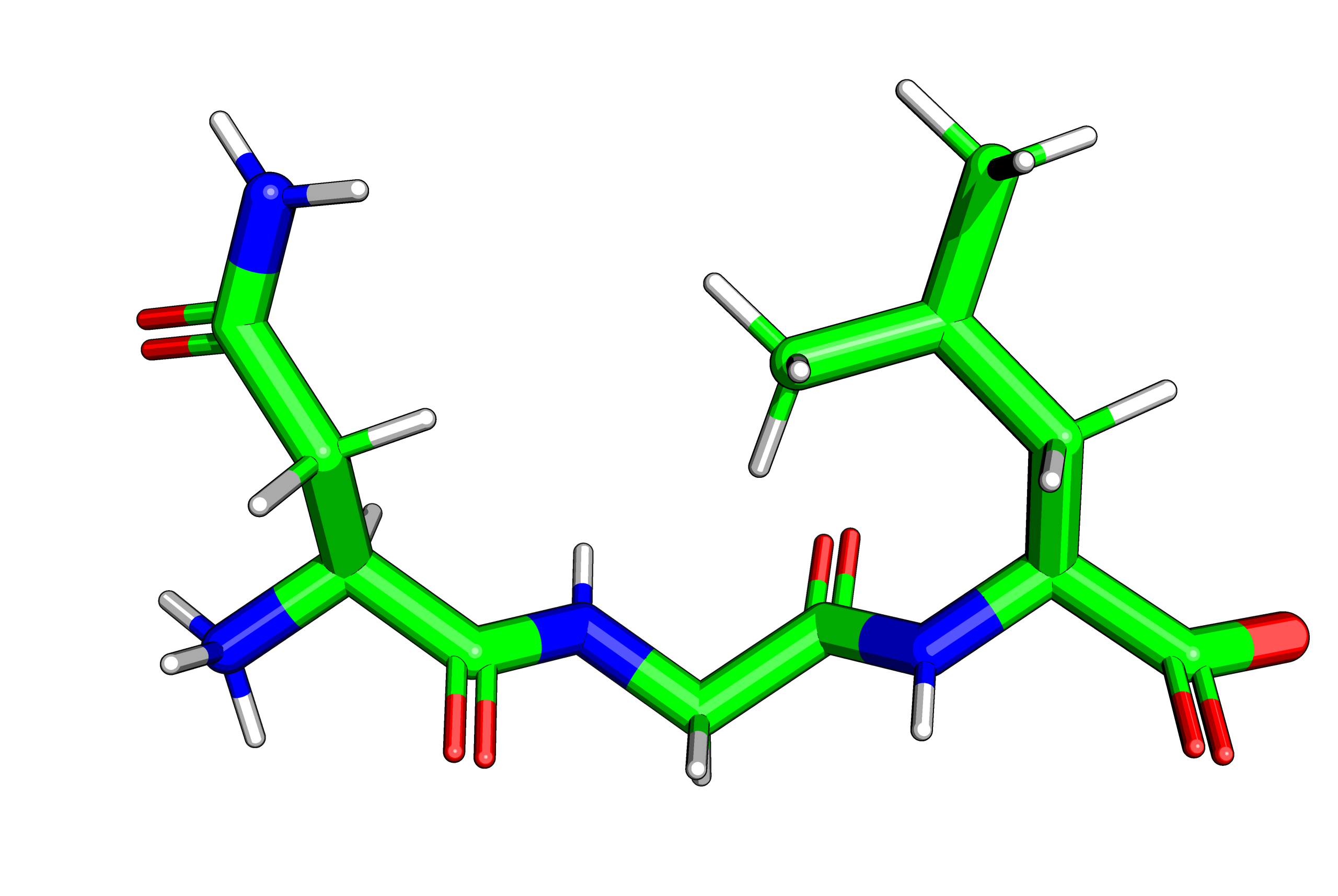
Michelanglo — VENUS
Variant effect on structure — Free energy
Energetics of protein destabilisation
Free energy of folding

The Gibbs free energy is the potential energy of the protein (technically a combination of entropy, enthalpy and temperature). The difference in energy of a folded protein relative to the unfolded state is simply referred to as ΔG. This is a negative value as the protein released energy to fold: so the more negative a the Gibbs free energy is the more stable a protein is. This energy is dependant on pressure and temperature. At a given temperature the ΔG is zero: this is the melting temperature of the protein where 50% is fold and 50% is unfolded (and the hypothetically uncrowded protein can return to a folded state).
Therefore a deleterious mutation will have a positive difference in ΔG (ΔΔG) and a lower theoretical melting temperature. A deleterious mutation, however, does not need to increase the ΔΔG all the way to zero to be deleterious in vivo: a sufficiently large local destabilisation of the structure is sufficient to cause the protein to aggregate and therefore be targetted to the proteasome.
The difference between wild type protein and a mutant is dictated by the strain on torsion angle of the atoms involved and by difference in non-covalent bonds.
The meaning of kcal/mol
The units used to describe the Gibbs free energy and other energies in biochemistry is kcal/mol. Although, like for Kelvin and Celcius, kJ/mol may be used, 4 kJ/mol are 1 kcal/mol. An often cited value is that of the C-H bond, which is 100 kcal/mol, or that of a peptide bond (20 kcal/mol), but protein folding does not break bonds, so this is misleading. In fact, the strength of the interactions involved are smaller:
- a hydrogen bond is between 1–3 kcal/mol
- a salt-bridge (e.g.lysine-glutamic acid) is about 4–5 kcal/mol
- a π-π interaction is between 2–3 kcal/mol
- a π-sulfur interaction is between 0.5-2 kcal/mol
- an alanine residues in a cis conformation has penalty of 3-7 kcal/mol ( ref )
The non-crystalline water surrounding the protein in the meanwhile is colliding and the average collision has 0.7 kcal/mol, as determined by multiplying the Boltzmann constant by the temperature (which for our discussion is 37°C).
Pyrosetta

Limitations
However, other properties impact on the behaviour of a protein, independently of its interactions. For example, the isoeletric point (pI) of a protein has profound effects on its solubility regardless of how stably it is folded. Specifically, a protein is less soluble (i.e. will aggregate) if the pI is close to the pH of its environment, supposedly pH 7.4. Another example, is the presence of a large hydrophobic path, which physiologically behaves differently than in similations of an isolated protein, namely by forming aggregates.
Further reading
For more see Chemical Bonds in Biochemistry in NCBI , protein folding in Wikipedia